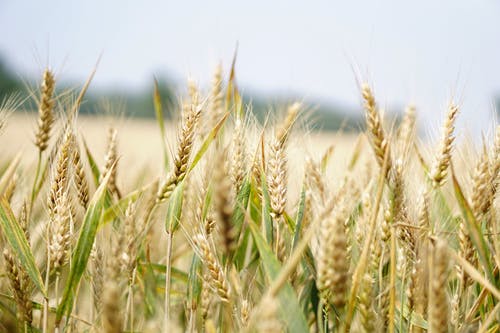
Nitrogen-Fixing Bacteria Are the Latest Genetic Engineering Breakthrough
By Henry Miller and Kathleen Hefferon
Much of the world is preoccupied with the ongoing coronavirus pandemic, but there are other global challenges, including climate change, food security, and degradation of the environment. Interestingly, and perhaps ironically, there is some good news regarding the latter three from a recent breakthrough in microbiology.
Plants depend upon beneficial interactions between roots and root-associated microorganisms for growth promotion, disease suppression, and nutrient availability. Crops require nitrogen to grow, and although there is an abundance of it in the atmosphere, it is not available in a form that plants can readily use. Instead, the roots of most crops take up nitrogen in the form of ammonia, either as synthetic fertilizer or in biological waste such as manure or compost. The availability of nitrogen from any of those sources increases crop yield, which is in itself an important step toward achieving food security.
Before synthetic fertilizers became available, feeding the world’s population relied solely upon an endless demand for manure to such an extent that wars have been fought over it. For example, in 1864 a naval conflict broke out between Spain and Peru over the Chincha Islands, which were covered in guano deposits said to be over 100 feet high.
Some plants take up nitrogen with the help of soil bacteria that actually “fix” nitrogen gas (N2) from the atmosphere, converting it into ammonia, which the plant’s roots can use. This process of biological nitrogen fixation (BNF) is tightly regulated by the bacteria that perform this action, so that the process is switched off if sufficient levels of nitrogen are available in a form that can be taken up by the plant. These microorganisms are found associated among the roots of certain leguminous crops, such as soybean, alfalfa or pea, which contain nodules within their root systems where these processes are carried out. Crops such as these can be rotated in the field with others which lack this ability, such as corn or wheat, for example, to obtain higher yields and for maintenance of soil health.
Synthetic fertilizers are now essential to feeding the world’s population, but they are costly and their production requires fossil fuels. While saving and enhancing the lives of countless people, synthetic fertilizers have had other, undesirable effects, especially on the environment. Excess nitrogen in the form of ammonia can make its way out of farmland and into our waterways where it collects and forms anoxic dead zones, such as the one found where the Mississippi River Delta meets the Gulf of Mexico. Another aspect of the problem is that excess ammonia left on farmers’ fields can also be converted into nitrous oxide (N2O), a stable, long-lived greenhouse gas with 300 times the potency of carbon dioxide. Nitrous oxide represents six percent of greenhouse gas emissions, about three quarters of which is derived from agriculture. In addition, N2O also diminishes the ozone layer, further exacerbating climate change.
A long-sought solution would be to replace synthetic fertilizers with bacteria that perform nitrogen fixation in the roots of crops other than legumes, which could reduce nitrogen runoff and the production of nitrous oxide. This has now been achieved, as described in an article published last December by a large group of academic and industrial researchers.
Their report described “the identification, development, and deployment of the first microbial product optimized using synthetic biology tools to enable BNF for corn (Zea mays) in fertilized fields, demonstrating the successful, safe commercialization of root-associated diazotrophs and realizing the potential of BNF to replace and reduce synthetic nitrogen fertilizer use in production agriculture.”
They demonstrated that a genetically engineered variety of the soil bacterium Klebsiella variicola will fix nitrogen and effectively create fertilizer for non-leguminous crops, such as corn. The bacteria would thus help these high-demand crops grow robustly and produce high yields with a reduced need for fertilizer inputs, and with less of their negative effects.
The bacterium chosen for this work already contained the genes that enabled it to fix atmospheric nitrogen via an enzyme called nitrogenase, so it comes by this ability naturally. However, because nitrogenase expression is switched off in the presence of ammonia, applying synthetic fertilizer would suppress bacterial BNF. By using a genome editing approach that employs CRISPR, the bacteria were tweaked to express the enzyme in the presence of fertilizer, which boosted the levels of nitrogen in a form available to the plant.
The study demonstrated that the need for applications of synthetic fertilizer was significantly less when this engineered bacterium was provided as an inoculum to the roots of non-leguminous crops such as corn. Most important, the authors showed that the system worked not just in the greenhouse but under a variety of real-world field conditions.
This elegant study could be a game-changer on many fronts. The process of generating synthetic fertilizer requires fossil fuel, and the simple act of applying it to farmers’ fields via tractor use burns even more fuel and thus exacerbates greenhouse gas emissions. Fewer applications of fertilizer (or manure) means fewer opportunities for nitrogen runoff and greenhouse gas emissions to be created, while maintaining the crop yields necessary to feed a burgeoning global population.
If the use of genetically engineered microorganisms could solve three of the major challenges in agriculture today, with no downside, who could possibly take issue with that? Unfortunately, there are numerous precedents for failing to embrace agricultural innovations. One egregious example was the saga of the genetically engineered “ice-minus” Pseudomonas syringae applied to plants such as strawberries to prevent frost damage.
The harmless wild-type bacterium, Pseudomonas syringae, which lives on many plants, contains an “ice nucleation” protein that promotes frost damage. (Ice nucleation proteins, which are found on the surface of certain bacteria, promote frost damage in plants by inducing the formation of ice crystals at a higher temperature than they would otherwise form.) In the early 1980s, scientists in the agricultural biotechnology industry and at the University of California, Berkeley, devised an ingenious approach to limiting frost damage, using recombinant DNA, or “gene-splicing,” techniques. They created a mutant of the bacterium that lacks the ice-nucleation protein, reasoning that spraying this variant bacterium (dubbed “ice-minus”) on plants might prevent frost damage by displacing the wild-type, ice-promoting variety. Using very precise recombinant DNA techniques, the researchers deleted the gene for the ice-nucleation protein and planned field tests with the ice-minus bacteria to see whether it would actually prevent frost damage under real-world conditions.
So far, so good. Then the government stepped in.
The Environmental Protection Agency (EPA) classified the innocuous ice-minus bacteria, which were to be tested in northern California on small, fenced-off plots of potatoes and strawberries, as a pesticide. The rationale was that because the naturally occurring, ubiquitous “ice-plus” bacterium promoted frost damage, it was, therefore, a “pest,” and other bacteria intended to mitigate its effects would be considered a pesticide. This is the kind of absurd, sophistic reasoning that could lead the EPA to regulate outdoor trash can lids as a pesticide because they deter or mitigate the actions of a “pest”—namely, raccoons.
At the time, scientists inside and outside the EPA agreed that the test posed negligible risk. The reasoning was that no new genetic material had been added—only a single gene whose function was well known had been deleted—and the organism was obviously harmless. Nonetheless, the field trial was subjected to an extraordinarily long and burdensome review, by both the National Institutes of Health and EPA, only because the organism had been genetically modified with recombinant DNA techniques.
It is noteworthy that small-scale field trials using bacteria with identical traits, but constructed with older, cruder techniques require no governmental review of any kind. (There are natural, ice-minus deletion mutants of P. syringae, but because the gene for the ice-nucleation protein is not completely deleted, the mutation isn’t permanent.) When field tested on less than 10 acres, non-engineered bacteria and chemical pesticides are completely exempt from regulation. Moreover, there is no government regulation at all of the vast quantities of the wild-type, “ice-plus” P. syringae organisms (which contain the ice-nucleation protein) that are commonly blown into the air during snow-making at ski resorts.
Although the ice-minus bacteria proved safe and effective at preventing frost damage in field trials, further research and commercialization were discouraged by the combination of onerous government regulation, the inflated expense of doing the experiments, the prospect of huge downstream costs, and the stigma of pesticide registration. As a result, the product was never commercialized, and plants cultivated for food and fiber remain vulnerable to frost damage.
The failure of the ice-minus P. syringae due to bureaucrats’ malfeasance might be thought of as a cautionary tale. In spite of the obstructionism of regulators and the opposition of activists, however, the innovations just keep on coming.
For years, farmers and scientists have been aware of a phenomenon known as “commensalism,” a long-term biological interaction in which members of one species gain benefits while those of the other species neither benefit nor are harmed; specifically, certain crops benefit from their intimate associations with their local soil microbial community. Thus, microorganisms are being developed for various agricultural applications, including as biopesticides; soil remediators to remove pollutants; and biosensors to detect crop stress or assess the microclimate of fields, orchards, and vineyards. The genomes of individual microorganisms as well as entire microbial communities are being examined using computational tools, including AI, to determine which can improve crop yield, reduce pest pressure, control weeds, and deliver nutrients.
For millennia, microorganisms have been used for fermentation of food and beverages, and, more recently, as a source of pharmaceuticals and industrial enzymes. They are truly part of the story of humanity. Using them to produce higher crop yields, which would feed more people at less expense, improve farmers’ bottom line, and reduce agriculture’s greenhouse gas footprint, is a logical and welcome extension.
Inevitably, however, there will be opposition to this innovation. The organic lobby, intransigent, anti-technology activists, and ignorant politicians won’t magically become enlightened. The recent fiasco in Sri Lanka, which tried to convert completely to organic farming, with disastrous results, is one example. Another is the European Union’s Farm to Fork strategy, which aspires to increase organic crop production 20% by 2030. Accomplishing that would require the conversion of more wild places into farmland, and a demand for compost, manure and other forms of biological waste that cannot possibly be met.
What will it take for policy makers to rethink their opposition to technologies such as genetically engineered plants and commensal microorganisms? Widespread famine? Astronomically inflated food prices? Extreme climate catastrophe? We hope not, but as with the sorry saga of nuclear power, history is not on our side.
Read More