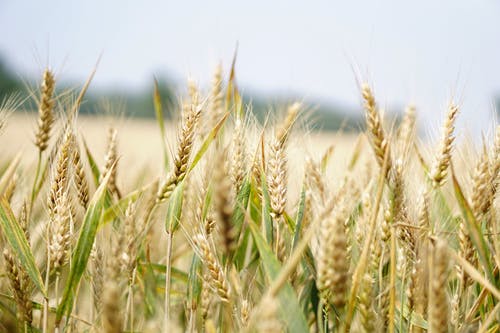
Canada poised to endorse crop gene editing
Gene editing, which allows precise edits to the genome, has been widely used for a variety of applications in laboratories worldwide since its discovery a decade ago. It has tremendous potential: Researchers hope to use it to alter human genes to eliminate diseases; improve the characteristics of plants; resist pathogens; and more. The two scientists who discovered the iconic gene editing technology, the CRISPR-Cas9 system, were awarded the 2020 Nobel Prize in Chemistry.
In spite of the fact that gene editing is essentially a refinement of earlier, less precise, less predictable techniques for genetic modification, finding the right approach to regulating it has been elusive. Initially, many nations treated it as a stringently regulated “GMO,” or “genetically modified organism,” which posed conceptual problems from the outset. For one thing, there’s really no such thing as a GMO, except in the fevered imagination of bureaucrats, legislators, and activists, but that didn’t prevent this pseudo-category from being subjected to onerous regulation.
Genetic engineering, or genetic modification, is a seamless continuum of techniques that have been used over millennia, including (among others) hybridization, mutagenesis, somaclonal variation, wide-cross hybridization (movement of genes across “natural breeding barriers”), recombinant DNA, and now gene-editing. The primary distinction between the last two and the others is they are far more precise and predictable than the earlier techniques.
Since the advent in the 1970s of recombinant DNA technology, which enables segments of DNA to be moved readily and more precisely from one organism to another, molecular genetic engineering techniques have become ever more sophisticated, precise, and predictable. This evolution has now culminated in the most recent discoveries, the CRISPR-Cas9 system and variations of it. It’s a way to find a specific bit of DNA inside a cell and then to alter that piece of DNA. CRISPR can also be used to turn genes on or off without altering their sequence.
CRISPR (short for Clustered Regularly Interspaced Short Palindromic Repeats) is a natural defense system that a range of bacteria use against invading viruses. CRISPR can recognize and guide the system to specific DNA sequences, while the enzyme Cas9 (or other Cas proteins) cuts the DNA at the recognized sequence. As often happens in science – and reminiscent of mutagenesis a century ago and recombinant DNA technology in the 1970s – molecular biologists and genetic engineers quickly copied and adapted the naturally occurring system. Using CRISPR-Cas9, scientists can target and edit DNA at precise locations, deleting, inserting or modifying genes in microorganisms, plants and animals, including humans. CRISPR-Cas9 is cheaper, faster, easier, more precise, and more predictable than its genetic engineering predecessors, and scientists are continuously improving the technique, its predictability and safety.
The US and Canada have deregulated gene-edited organisms in principle, moving towards risk-based regulation, while Europe, with its long-standing, intractable opposition to genetic engineering, has decided to equate gene editing with heavily regulated, and sometimes even banned, GMOs. Other nations, such as the UK, are beginning to move away from Europe’s hostile regulatory climate, with hopes of improving their farmers’ livelihoods as well as finding new partners for international food trade, besides their European friends.
The polar extremes of acceptance of genome editing in different countries appear to be a reflection of a social transformation around food which values “natural” products. Some have argued that genome editing should be distinguished from other new agricultural technologies such as the generation of transgenic plants by recombinant DNA (“gene-splicing”) techniques. This argument may be based on the fact, as mentioned above, that with genome editing, only a few nucleotides of a plant genome sequence may be altered (and, therefore is more “natural”), while transgenesis introduces genes from other species, such as viruses, bacteria, or eukaryotes. As such, the discussions of these issues become almost theological in nature, not unlike debates over how many angels can dance on the head of a pin, rather than based on science.
Some observers believe that the concept of “cisgenesis” (as opposed to “transgenesis”) could be a way to assuage or minimize the concerns that some people have about genetic engineering with the newer, more precise techniques. It refers to the genetic engineering of a recipient plant with genes from a crossable—sexually compatible—plant. The process adds no new genes or sequences not found in a compatible plant, and also absent are all selectable marker sequences such as antibiotic resistance or luciferase (which makes a recipient “light up”), whose presence in transgenic plants is often problematic for anti-GMO activists. Cisgenesis is sometimes proposed as a way to accomplish “rewilding,” that is, reintroducing into crop varieties desirable properties such as resistance to pathogens or drought present in wild relatives.
History is instructive. Humans have been selecting and breeding to introduce or enhance desirable traits such as yield or taste for millennia, but because of the imprecision of the techniques, this has often led to various beneficial wild genes eventually, inadvertently being bred out and lost over time. Precision and predictability are important to ensure that the results are safe and achieve their desired ends. There are notable historical examples of the use of older, pre-molecular techniques of genetic modification in agriculture that turned out to be problematic. Examples include the Lenape potato, which contained elevated, harmful levels of a plant alkaloid; the creation of hyper-aggressive Africanized honeybees by crossbreeding African and European species in the 1950s; and inadvertently causing some varieties of corn in the United States to become more susceptible to the Southern Corn Leaf Blight fungus, which resulted in significant crop losses in 1970.
We emphasize that cisgenesis is a result, not a technique or technology. Only selected genes are introduced into the cultivar, but not unwanted genes that may be responsible for toxicity or other undesirable traits such as bad taste or lower yield. The easiest way by far to accomplish this is by the use of molecular techniques, such as recombinant DNA technology or gene editing.
If plant breeders try to rewild crops using conventional approaches, they are often faced with linkage drag, in which unwanted, sometimes deleterious genes get passed along with the desired trait. That then requires successive generations of recurrent backcrossing and simultaneous selection to create a cultivar in which the gene of interest is no longer linked to any undesirable genes. This can be a long and slow process, taking many years, depending upon how tightly linked the genes are and the generation time of the plant.
By contrast, cisgenesis isolates only the gene(s) of interest from the donor wild plant, which makes it possible to produce disease-resistant trees, such as apples resistant to fire blight fungus disease, for example, or potatoes resistant to late blight disease. It is particularly appropriate for the lengthy process of tree breeding, as well as for producing vegetative crops such as grape, potato, or banana. Cisgenesis can also facilitate the stacking of resistance genes from several sexually compatible plants, in order to introduce resistance to multiple threats. Also, because the sequences introduced into cisgenic crops are derived from plants that are sexually compatible, the resulting plants are indistinguishable from their traditionally bred counterparts. They contain no foreign sequences, thanks to the use of enzymes that remove selectable marker genes.
Research studies of European consumers’ acceptance of cisgenic crops have been encouraging, and some have argued that if the “category” of cisgenic crops, whatever the techniques used to craft them, were deemed acceptable for cultivation by European regulators, that could cause a paradigm shift in regulation. In other words, cisgenics would be a kind of Trojan Horse, opening the way to future deregulation. Thus, there is a movement in some quarters to regulate cisgenic plants crafted with molecular techniques no differently than similar plants made with conventional techniques. Health Canada is expected to announce that policy any day. But even with our current state of knowledge about the seamless continuum of techniques of genetic modification with respect to risk, by regulating gene-edited crops like conventionally modified ones, their food regulators create a meaningless distinction between cisgenic gene editing and transgenic, recombinant DNA modifications.
We find that approach flawed and unpersuasive, because it is unscientific. The mere fact of transgenesis — the process of introducing a transgene (i.e. an exogenous gene) from one organism into another so that it exhibits a new, heritable property — is unrelated to risk. Moreover, genomic sequencing has revealed that extensive DNA changes occur during conventional breeding, and that some plants such as sweet potato have, over time, incorporated into their own genome fragments of DNA from the pathogenic microorganism Agrobacterium and are thus, in effect, “natural GMOs.”
Simply stated, whether it encompasses gene editing or not, “GMO” is an arbitrary and meaningless “pseudo category,” and regulating it more stringently than conventional breeding makes no sense. Once we spurn science as the basis for regulatory policy, we’ve relegated ourselves to a game of, “How stupid and irrational do we intend to be?” In other words, where is the limit on accepting false assumptions?
The science tells us that there is a seamless continuum of genetic modification processes — from the natural selection that occurs as the result of Darwinian evolution, including the “natural GMOs” alluded to above; selection and breeding; mutagenesis; somaclonal variation; “wide crosses”; recombinant DNA; and gene editing. But many national regulatory agencies continue to ignore this and impose oversight regimes that discriminate against the use of the most precise and predictable techniques, exactly the opposite of what risk analysis dictates. Although a strategy of favoring cisgenics might result in some genetically engineered plants being cultivated in Europe and other countries with stringent regulation of those superior techniques, it would further stigmatize transgenic crops. It would represent expediency over principle.
How do we resolve this regulatory conundrum? There have, in fact, been workable models of scientifically defensible, risk-based approaches to regulation (such as here and here), but they have not been widely or comprehensively adopted. Even so, we take the long view — that sound science must prevail in crafting regulatory policy. Many of us in the scientific community will settle for nothing less. Forsaking science does not end well.
Henry Miller, a physician and molecular biologist, is a senior fellow at the Pacific Research Institute. He was a Research Associate at the NIH and the founding director of the FDA’s Office of Biotechnology. Find Henry on Twitter @henryimiller
Kathleen Hefferon, Ph.D., teaches microbiology at Cornell University. Find Kathleen on Twitter @KHefferon
Read More